Molecular Motors in Fluidic Channels
Overview
Cells use active transport mechanisms for the directional intracellular movement of materials. Microtubules, long hollow cylinders made of the protein tubulin, serve as rails for the transport of cargo. Biomolecular motors, such as kinesin, can walk along these tracks transporting a cargo while using the energy of ATP hydrolysis. Kinesin is a dimeric motor protein that moves processively along a microtubule in 8 nm steps.
The movement of kinesin along microtubules can be reconstituted in cell-free conditions. In an inverted gliding assay the kinesin motors are fixed to a substrate, and movement of microtubules along the kinesin-coated surface can be observed (movie). The direction of movement is completely arbitrary.
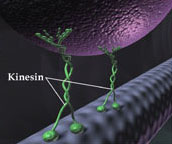
In vivo kinesin (B. Pletsch 2003, MCG)
Goal of our research
Inspired by nature, we want to explore the use of kinesin motor proteins actively transporting microtubule shuttles in engineered environments. The energy derived from ATP hydrolysis can be harnessed to perform work on the nanoscale for possible purposes as molecular sorting, transporting or actuation.
Possible merits of motor-driven transport are:
- Active transport beyond diffusion
- Cargo loading can be specific and selective
- Work against fluid flow
In a functional molecular motor-driven transport system one has to be able to exert control over various aspects of the system at a molecular level, for example the controllable placement of motors at designated areas, velocity of the shuttles, cargo loading, steering etcetera. In our project we work on the following components in the toolbox of molecular motor-driven transport:
- Confinement; microtubules need to be confined to certain paths/tracks
- Directionality; in applications, such as sorting, it is important to control unidirectional motion of microtubules in their tracks.
- Docking; it is important to have control where microtubules dock to their tracks, for example to pick up cargo at a specific location.
Confinement
We use nanofabrication techniques in combination with specific chemical absorption to create gold tracks for microtubule motility, recessed in silicon oxide substrates. Using e-beam lithography and dry etching we fabricate 1 μm deep tracks in the substrates. Gold is deposited on the trench bottoms by e-beam deposition. A self-assembled monolayer is adsorbed onto the surrounding SiO2 to block motility outside the gold tracks. Kinesin is adsorbed onto the gold tracks on the bottom of the trenches and microtubules can then be imaged while moving through the trenches. The trench sidewalls guide and confine the movement of microtubules.
Microtubules (red) moving over kinesin-coated gold tracks (green). A self-assembled monolayer on the elevated silicon oxide plateaus (black) blocks motility in those regions, effectively confining motility to the gold tracks. The movement of the microtubules is guided by the trench sidewalls. The images were made by overlaying a fluorescence image of rhodamine labeled microtubules with an optical picture of the nanostructures.
Scanning Electron Microscope image of a rectifier structure, showing clearly the geometry of the nanostructures. The image was taken under an angle, and the substrate was broken to show the cross section. Kinesin absorbs only on the gold (Au) areas, since the silicon oxide (SiO2) sidewalls and elevated regions are blocked by a self-assembled monolayer.
Optical image of nanostructures fabricated for guidance and confinement of microtubules.
Directionality
In an inverted gliding assay on a glass substrate, microtubules move in random directions over the substrate. In a molecular motor-driven transport system, the motion of microtubules has to be effectively guided, confined and directed. In particular, the rectification of microtubules will be important in applications such as molecular sorting, where it is envisioned that microtubules approach a Y-junction unidirectionally. Using nanofabrication techniques we have fabricated rectifier geometries that very effectively redirect microtubule movement into the desired direction. We have obtained a rectifier efficiency of 92%, as a result of which we could obtain that 97% of the microtubules in a trench moved unidirectionally.
Microtubules (red) traversing a rectifier geometry. Microtubules entering from the upper entrance are very effectively redirected in the rounded areas in the bottom of the geometry. Microtubules that enter from the lower entrance should pass unhindered and exit through the upper channel.
The images were made by overlaying a fluorescence image of rhodamine labeled microtubules (red) with an optical picture of the nanostructures (green). The green gold area is coated by kinesin molecules. The elevated silicon oxide regions (black) are blocked for motility.
<Click image for quicktime movie>
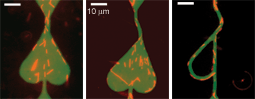
Fluorescence images of microtubules moving in random directions over a glass substrate.
Docking
When scaling down the size of the tracks for microtubule motility, there is a need for a more efficient docking of microtubule shuttles into the structures. Using electric fields, we demonstrated the electrically controlled docking of microtubules onto gold nanostructures. The use of nanofabrication techniques allows an easy integration of gold electrodes inside the microtubule tracks, thereby enabling control of shuttle docking at specific places at a substrate. In such a way, we have a local and temporal control over microtubules.
Without active control of microtubule docking, one needs a large area to absorb microtubules from the solution and feed them into the network of tracks. The scanning electron microscope image shows such a collection area, leading into a circular track for motility.
Fluorescence movie of microtubules docking into a gold microstructure using electric fields. Upon applying a voltage to the square gold electrode, microtubules are driven towards the surface and the density of moving ones increases. After switching of the voltage, the number of microtubules decreases again. The time evolution of the density of microtubules can be described by a simple equilibrium model.
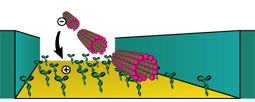
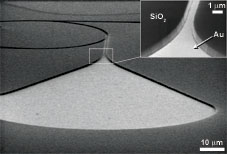