Synthetic cells on chip
We aim to establish an artificial life cycle of liposomes specifically exhibiting two fundamental characteristics of living systems: growth and division. We intend to do this on a microfluidic chip, where the liposomes are produced, grown, and finally divided, whereupon the cycle is repeated.
We have developed a novel microfluidic method, Octanol-assisted Liposome Assembly (OLA), which produces cell-sized (5–20 µm), monodispersed, unilamellar liposomes with an excellent encapsulation efficiency. OLA is a process akin to bubble-blowing which uses 1-octanol as the lipid-carrying solvent, and results in spontaneous formation of liposomes, along with the waste product, 1-octanol droplets. We further perform on-chip density-based separation of droplets, in order to get a pure yield of liposomes. These liposomes are further manipulated according to the need, such as immobilization using microfluidic traps or shape manipulation by squeezing them into narrow confinements.
We recently succeeded in inducing liposome growth by recruiting lipids from the external environment. We achieved this by membrane fusion of small unilamellar vesicles to the stressed membrane of mother liposomes. We have already shown that, by colliding the liposomes against well-defined microfluidic obstacles, we can split the liposomes into two, symmetric daughter liposomes, with negligible leakage.
Integrating these modules to establish a continuous life cycle of vesicles is a future challenge. This will provide a dynamic system of growing-and-dividing liposomes in an evolutionary cycle which can be used as a fundamental tool to apply the bottom-up strategy to synthetic cells.
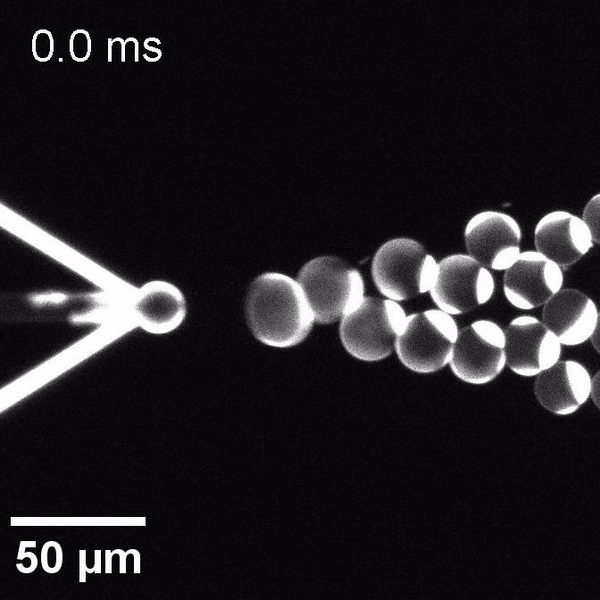
The controlled bubble-blowing process of Octanol-assisted Liposome Assembly (OLA), where monodispersed double-emulsion droplets are pinched off at the production junction to ultimately form unilamellar cell-sized liposomes.
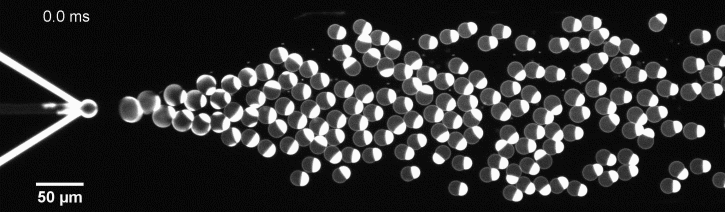
Probing biomolecular condensates as synthetic cells
Along with membrane-bound organelles, living cells contain numerous membraneless organelles, nowadays categorized as biomolecular condensates. They are a result of liquid–liquid phase separation, in particular complex coacervation resulting from electrostatic interactions between two or more oppositely charged polyelectrolytes. These condensates play a vital role in regulating intracellular biochemistry, and their dysfunction is associated with several protein-aggregation diseases. In order to study their dynamics, we recently developed an on-chip methodology to form coacervates inside cell-sized (~10 µm) liposomes. Protein-pore-mediated permeation of small molecules into liposomes allows us to trigger the phase separation process. We demonstrated sequestration of proteins (FtsZ) and supramolecular assemblies (lipid vesicles), as well as the possibility to host metabolic reactions (-galactosidase activity) inside these condensates. Our platform provides a versatile tool to understand intracellular phase behavior, and these hybrid systems may further facilitate bottom-up creation of synthetic cells.
Recent theoretical work has demonstrated the possibility of repetitive cycles of growth and division of coacervate-like systems, by maintaining them in a chemically driven non-equilibrium state. We aim to achieve such a growth-division cycle experimentally. We plan to produce coacervate droplets in microfluidic chips and keep them out-of-equilibrium by feeding them with individual components and at the same time triggering a decomposition reaction within them. This degradation of components will be explored through various protein systems, mainly through enzymes. A right balance of growth and decomposition will potentially induce a shape instability, leading to a grown coacervate dividing into two/more daughter coacervates.
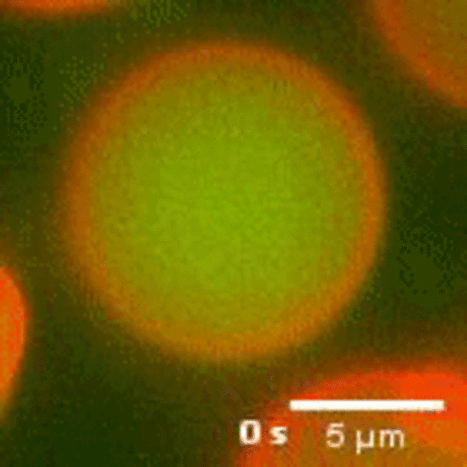
Controlled coacervate formation inside liposomes.
People working on this project
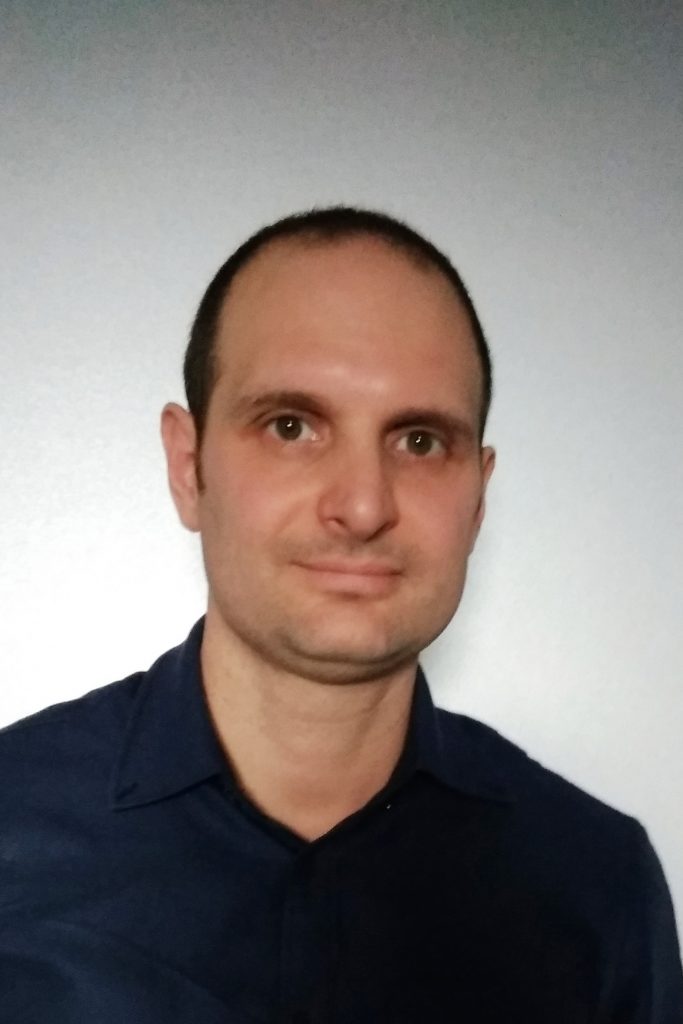
Nicola De Franceschi
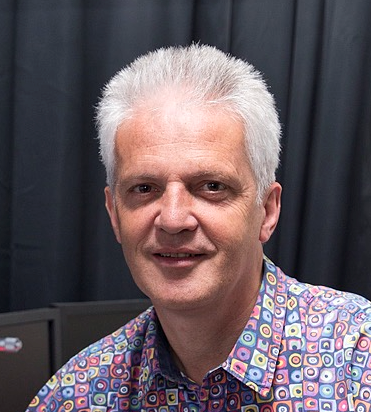
Cees Dekker
- F0.210
- +31-(0)15-27 86094
- C.Dekker@[REMOVE THIS]tudelft.nl
- Principal Investigator
- View CV
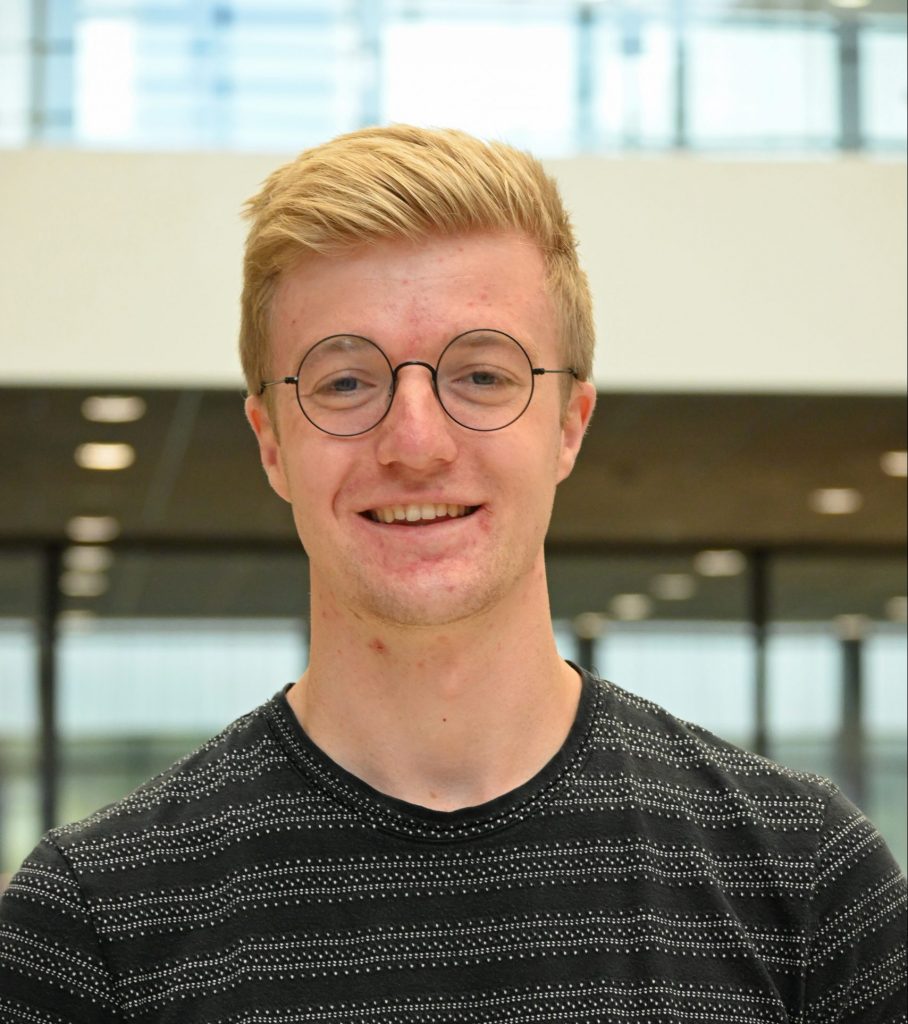
Bert van Herck
- F0.110
- B.VanHerck@[REMOVE THIS]tudelft.nl
- Research: Microfluidics and synthetic cells